By Alexis Adams, M.Sc., P.Ag.
Phosphorus is an essential nutrient for pulse crop productivity, and an adequate amount of phosphorus must be supplied in a plant-available form at the critical demand periods, to maximize crop production. Phosphorus management practices that minimize environmental damage and maximize economic returns for farmers are essential to maintain sustainable agroecosystems. The practices of using the right rate, right source, right timing, and right placement are known as 4R nutrient stewardship. 4R nutrient stewardship practices will promote long-term environmental, economic, and social sustainability. 4R phosphorus use practices in pulse crops give farmers and agronomists strategies to make best use of resources and maximize pulse crop production.
Understanding Phosphorus
Role of Phosphorus in Pulse Crops
Phosphorus is one of 16 essential plant nutrients and one of four macronutrients required in large amounts to sustain pulse crop growth. Phosphorus is a key component of molecules necessary for root growth and development, respiration, photosynthesis, nucleic acid synthesis, nitrogen fixation, plant maturity, and seed production (Zhang et al., 2014; Niu et al., 2013; Grant and Flaten, 2019).
Phosphorus is a component of several energy transfer molecules, including ADP, ATP, NADP, and NADPH, which provide energy for photosynthesis and other metabolic processes (Grant and Flaten, 2019, Zhang et al., 2014).
Phosphorus is also a component of DNA and RNA, chromosomes, genes, coenzymes, phosphoproteins, and phospholipids, all of which are necessary for cell function and plant growth and development (Grant and Flaten, 2019).
Phosphorus is required in greater amounts for pulse crops than many other crops due to its high demand in the energy transfer molecules used in nitrogen fixation (Rotaru and Sinclair, 2009). As phosphorus is a key component for many aspects of legume growth, agronomists and growers must take care to supply the crop’s phosphorus needs to maximize crop production.
Soil Phosphorus Cycling and Plant Uptake
Phosphorus exists in the soil in several organic and inorganic forms in soil organic matter, minerals, and in the soil solution (Figure 1). Plants take up phosphorus as orthophosphate ions (H2PO4– or HPO42-) from the soil solution. To maintain equilibrium, phosphorus moves from more available organic and inorganic pools to the soil solution (Grant and Flaten, 2019).
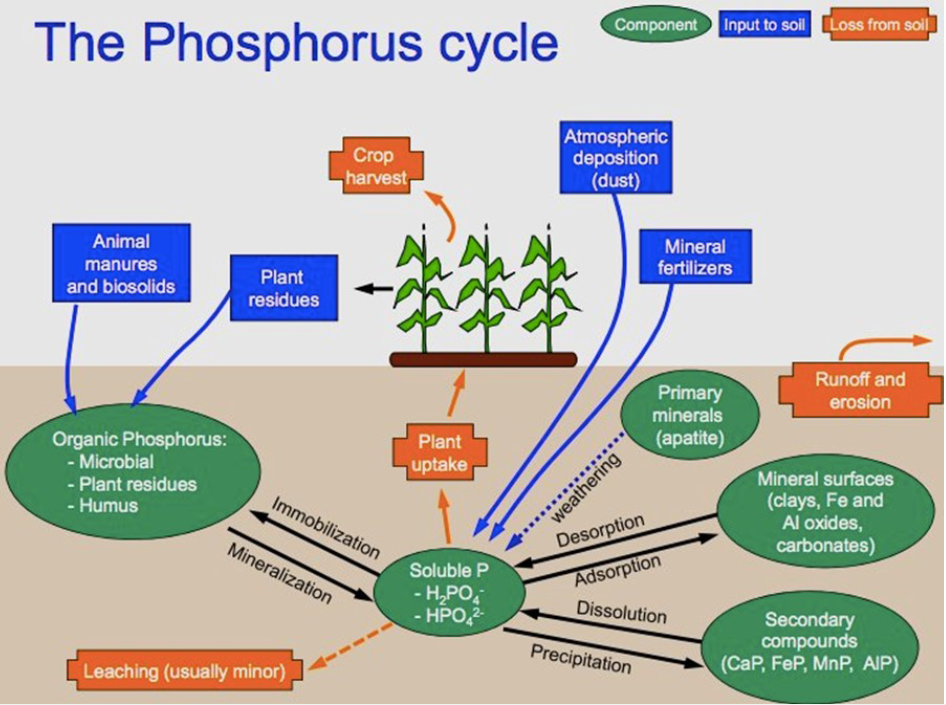
This more available, or labile phosphorus includes mineralizable organic phosphorus, readily exchangeable adsorbed phosphorus, and soluble forms of precipitated phosphorus. Phosphorus then moves from less available, or non-labile phosphorus pools, which include stable organic phosphorus and is strongly adsorbed to soil minerals and compounds, into the labile pool to maintain equilibrium (Figure 2) (Grant and Flaten, 2019). Phosphorus reacts strongly with calcium at higher soil pH levels and with iron and aluminum at lower pH levels. Because of the many ways that phosphorus interacts with soil particles and compounds, it moves extremely slowly through the soil.
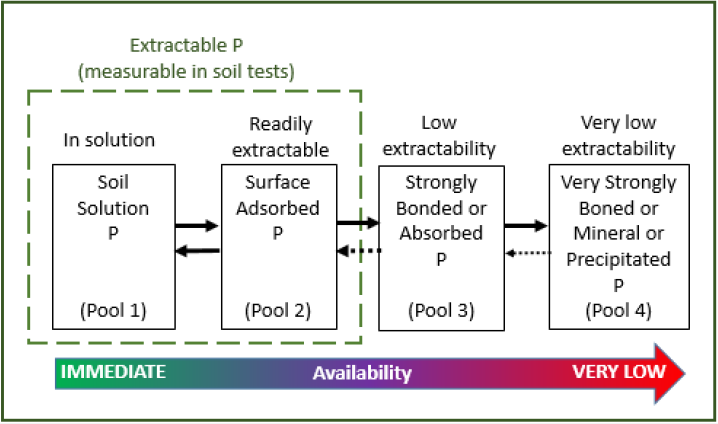
Phosphorus will not readily move through the soil towards plants, thus plant uptake is dependent on the presence of phosphorus in the soil solution, and roots in close proximity to it (Grant and Flaten, 2019; Syers et al., 2008). For continued phosphorus uptake, the soil solution of the root zone must be continually replenished with it.
When phosphorus fertilizer is added to the soil, the fertilizer granule attracts water, which dissolves the granule releasing orthophosphates into the soil solution for plant phosphorus uptake. Excess phosphorus not taken up by plants will move from the soil solution to labile and non-labile pools through several processes including precipitation, adsorption, and microbial immobilization.
Because of the many soil reactions phosphorus may undergo, it is relatively immobile in the soil, and a crop will only use about 25% of applied fertilizer phosphorus in the year of application. This may be discouraging to growers, however the applied phosphorus is retained in the soil and will be available to subsequent crops. When phosphorus applications are lower than crop requirements, the non-labile and labile phosphorus soil pools will be drawn on for plant uptake (Liu et al., 2015; McKenzie et al., 1992a; McKenzie et al., 1992b; Syers et al., 2008). With continually low phosphorus additions, however, soil reserves will be depleted, with limited phosphorus available for crop growth.
Plant Phosphorus Movement, Acquisition, and Deficiency
Plants live off of phosphorus stored within the seed during the process of germination and the first few days of plant growth. Once plant roots have begun to grow, phosphorus uptake begins. A small portion of plant phosphorus is metabolically active in energy cycles involving ATP and ADP. The rest of the plant phosphorus is stored in the plant as phytic acid or polyphosphate or stored as orthophosphate in cell vacuoles. When soil phosphorus availability is low, the plant will draw on this stored plant inorganic phosphorus (Ozanne, 1980).
When soil phosphorus is limited, plants attain more soil phosphorus through using acquisition strategies such as increasing root surface area through increased root hairs, release of root exudates to acidify and solubilize phosphorus, recruiting phosphorus solubilizing arbuscular mycorrhizal fungi (AMF) to colonize roots, and/or increasing the activity of phosphorus transporters (Sandana and Pinochet, 2014).
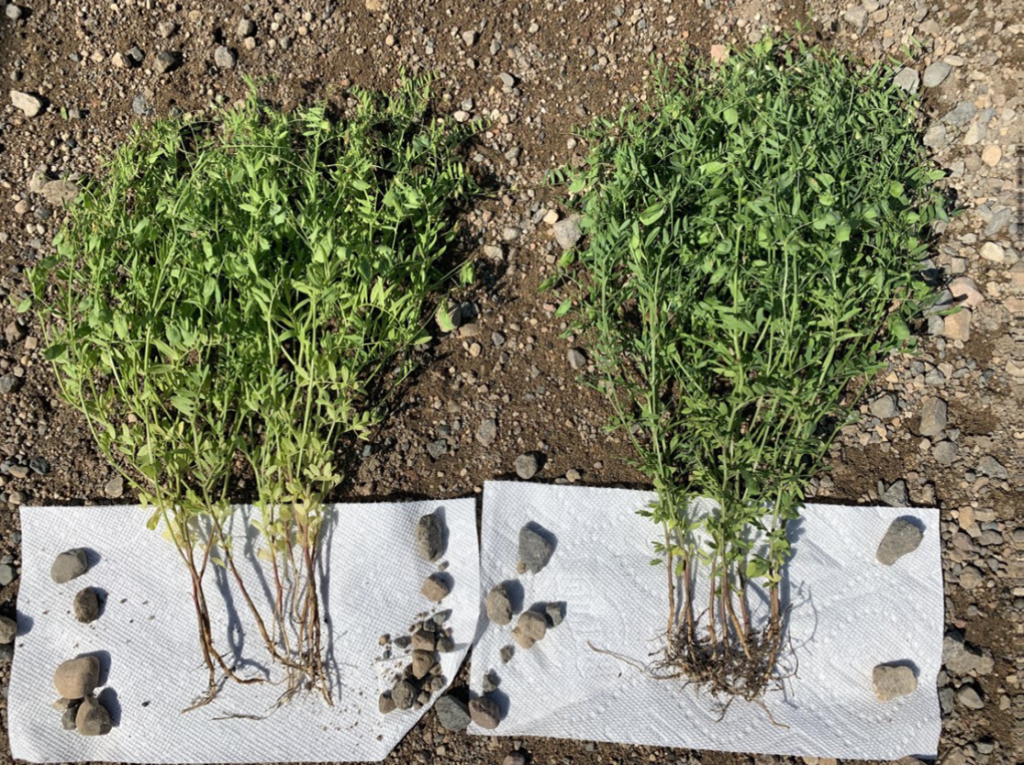
Source: Andrew Reddekopp
Generally, phosphorus deficiency symptoms in pulse crops include delayed emergence, delayed development of plants or leaves, stunted plants, thinner stems and roots, increased root to shoot ratio, reduced nodulation, lower yield, leaf colour change (dark green, yellow, purple, or brown), and senescence of oldest leaves (Hopkins, 2015; Hoppo et al., 1999, Sarker et al., 2015; Nielsen et al., 2001). Darkened green leaves are a result of accumulation of carbohydrates from decreased plant respiration. Leaf purpling is a result of anthocyanin accumulation due to a blockage of metabolic pathways from a lack of the necessary phosphorus-containing molecules (Close and Beadle, 2003). Phosphorus is mobile in plants, thus leaf colour change will appear first in the oldest plant tissue, as phosphorus is transported to growing points. As phosphorus deficiency progresses, metabolic activities in the oldest leaves shut down, and the leaves prematurely senesce and fall off of the plant.
In peas, phosphorus deficiency may result in reduced branching as well as darkened plants with red stems, tendrils, petioles, or leaf margins. In faba beans and lentils, the oldest leaves of phosphorus-deficient plants may have mildly mottled yellowing, along with purpling within dark green spots on chlorotic leaves. Chickpeas under phosphorus-deficient conditions will also be stunted and turn dark green, followed by interveinal leaf chlorosis, with leaf purpling in some cases. Phosphorus deficiency in dry beans, similarly to other pulse crops, results in slow growing, stunted plants with thin stems. Younger dry bean leaves are small with darker green pigmentation, and older leaves are chlorotic and browned and eventually die off. In soybeans, phosphorus deficiency causes stunted plant growth and appears as dark green or bluish leaf coloration, with complete or mottled interveinal chlorosis and purpling or browning within chlorotic regions.
4R Management Principles for Phosphorus
1) Right Source
Phosphorus can be applied in several forms, from several sources. The most accessible and commonly applied source is commercial fertilizer, of which there are several products and some special formulations. Other sources include rock phosphate, animal manure, and reclaimed waste products. As well, fertilizer additives, polymer coatings, and microbial products have been developed with hopes of improving phosphorus availability and uptake.
A) Mineral and Commercial Sources of Phosphorus Fertilizer
Commercial phosphorus fertilizer is derived from phosphate rock, which is also available for application as an unprocessed product. The phosphorus in phosphate rock is present in its mineral form, mainly bound to calcium, and has low plant availability. The mineral structure, particle size, and source of the rock phosphate being applied impacts the ability of the phosphorus to dissolve into the soil solution (Grant and Flaten, 2019). Solubility of rock phosphate also decreases in basic soils and with increased soil calcium content. Saskatchewan soils are generally both basic and calcareous, thus rock phosphate is not recommended as an immediate source, however it may be suitable as a long-term source for building soil phosphorus. Pulse crops acidify their rhizosphere to solubilize phosphorus and thus may do a better job of accessing rock phosphate than other crops (Bekele et al., 1983).
Rock phosphate is processed to form commercial fertilizer, which is a more pure and readily available source. The most commonly used dry commercial phosphorus fertilizer in Western Canada is monoammonium phosphate (MAP, 11-52-0, NH4H2PO4). MAP dissolves readily into ammonium (NH4+) and phosphate (PO43-) in water. As plants take up NH4+ ions, H+ ions are released to the soil solution, which decreases soil pH, preventing precipitation of the phosphate ions, which takes place at higher pH levels (Miller et al., 1970). The most common liquid source of phosphorus is ammonium polyphosphate (APP, 10-34-0, (NH4H2PO3)n(OH)2). As with MAP, the ammonium (NH4+) ions in this product lower pH and improve phosphorus uptake (Grant and Flaten, 2019). A less common source of phosphorus fertilizer is diammonium phosphate (DAP, 18-46-0, (NH4)2HPO4–). DAP is less suitable for Western Canadian pulse crops as it has lower seedling safety especially in calcareous soils, and is less acidifying than MAP and thus precipitates more readily in high pH soils (Bouldin and Sample, 1959).
Other specialty formulations of phosphorus such as Mosaic’s MES-15 (13-33-0-15), Koch’s 12-45-0-5, and Simplot’s 16-20-0-14 combine nitrogen, phosphorus, and sulphur in one granule. MES-15, a combination of MAP, ammonium sulphate (AS), and elemental sulphur is thought to improve phosphorus uptake because the ammonium (NH4+) in the AS reduces soil pH, and the sulphate ions precipitate with calcium in place of phosphate. Growth chamber and field research in canola, however, found no difference in phosphorus uptake between AS applied with MAP and MES-15. MES-15 was, however, found to have better seedling safety than AS+MAP at the same rates (Grenkow, 2013). Alpine is a company that produces several liquid nitrogen, phosphorus, potassium fertilizer blends. In comparison to liquid APP, a larger percentage of the phosphorus in Alpine is in the orthophosphate form than the polyphosphate form, and as such is thought to be more readily plant available.
B) Reclaimed and By-Product Phosphorus Sources
By-product and reclaimed sources of phosphorus such as manure and struvite have potential to improve farm sustainability through recycling nutrients. Animal manure contains both inorganic and organic sources of phosphorus, and when available in a reasonable distance from a field, is an economical source. Manure, however, is a source of nitrogen, thus growing pulse crops in the same year as manure addition may inhibit nitrogen fixation. Manure applied at rates matching nitrogen uptake of Western Canadian crops will typically leave phosphorus in the soil to benefit pulse crops grown later on in the rotation.
Struvite is a phosphorus source derived from municipal wastewater and/or animal manure, which shows potential for nutrient recycling and provides an alternative source to non-renewable phosphate rock (Ackerman et al., 2013). Struvite is formed through the addition of magnesium to wastewater, which causes precipitation of nitrogen and phosphorus into pearls. In this process approximately 50% of wastewater nitrogen and 90% of wastewater phosphorus contents are recovered (Rahman et al., 2014). Struvite has been found in research to perform well in soils with high and low pH (Massey et al., 2007). Struvite is reported to have similar phosphorus uptake and dry matter yield to commercial fertilizers in some research (Gonzalez Poneer and Garcialopez, 2007; Johnston and Richards, 2003), but has received limited field evaluation in pulse crops in Western Canada.
C) Phosphorus Additives and Coatings
Maleic-Itaconic Co-Polymer additives, such as Avail, are promoted to form complexes with calcium, iron, and aluminum surrounding the phosphorus fertilizer granule. Complexed calcium, iron, and aluminum are then unable to react with phosphates, allowing phosphorus to remain in the soil solution (Grant and Flaten, 2019). The most benefit for this co-polymer appeared to be where soil test phosphorus was low, rate of applied phosphorus was low, and at extreme pH levels (Hopkins et al., 2018).
Phosphorus may also be sold as a polymer-coated granule. The idea behind polymer coatings is to slow the release of phosphorus fertilizer by slowing the rate of dissolution and providing available phosphorus throughout the growing season. Polymer breakdown rates in the soil increase with increasing temperature and moisture (Du et al., 2006). Field research in Manitoba found polymer-coated MAP did not improve yield over regular MAP (Grant, 2002), however, polymer-coated phosphorus was found to improve seed safety of MAP in a plot study of 10 different crops (Schoenau et al., 2007).
D) Microbial Products
Microbial products such as Penicillium bilaiae and Arbuscular Mycorrhizal fungi (AMF) have also been marketed to improve soil phosphorus availability and plant uptake. P. bilaiae is sold commercially as an inoculant and seed treatment. P. bilaiae has potential to improve availability and uptake by secreting phosphorus-solubilizing organic acids that decrease pH and bind with calcium phosphate minerals to release phosphorus (Knight et al., 2011). Western Canadian research has shown mixed results across several annual crops from no response, to increased root growth and tissue phosphorus concentration, with some yield responses (Grant et al., 2002; Karamanos et al., 2010; Mohr et al., 2013; Vessey and Heisinger, 2001, Gan et al., 2003). Crops grown on soil with higher pH levels and calcium phosphate content will likely show a greater benefit from P. bilaiae application.
Arbuscular mycorrhizal fungi (AMF) is a naturally occurring soil microbial organism that has been researched as a soil amendment to improve plant uptake of phosphorus.
Crop roots form symbiotic relationships with AMF in which the plant provides photosynthates to the AMF and the AMF draw in nutrients beyond the root depletion zone through fungal hyphae (Grant et al., 2005). Pulse crops are dependent on AMF associations for nutrient acquisition, however, research has shown mixed response to additions of AMF amendments, especially under field conditions. (Xavier and Germida, 1997; Holzapfel et al., 2015; Knight et al., 2011). AMF amendment is most likely to provide a yield benefit in pulse crops grown after non-AMF host crops such as canola (Grant and Flaten, 2019; Holzapfel et al., 2015), under conventional tillage (Monreal et al., 2011; Grant and Flaten, 2019), or in low phosphorus soils (Grant et al., 2005). There may be potential for microbial products such as P. bilaiae and AMF to improve phosphorus acquisition, however research results have been mixed, and effectiveness is likely highly dependent on soil conditions.
2) Right Rate
A) Phosphorus Rate Strategy
There are two strategies for selecting phosphorus fertilizer rates, the short-term sufficiency strategy and the long-term sustainability strategy. The short-term sufficiency strategy involves applying only the amount of phosphorus that would elicit a crop response in the year of application. The long-term sustainability strategy, contrarily, involves replacing phosphorus removed by crop harvest over time to maintain soil levels (maintenance) or applying beyond crop requirements to increase soil phosphorus (building) (Malhi et al., 1993; McKenzie et al., 2003; McKenzie et al., 2001) (Figure 4).
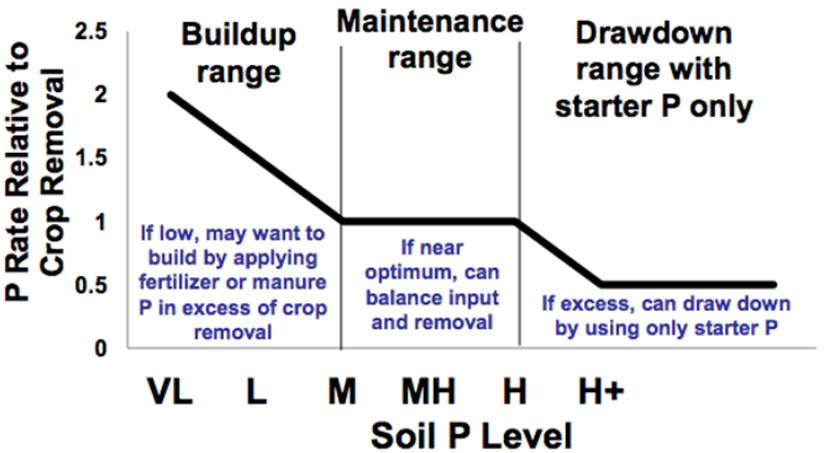
B) Soil Testing Importance and Soil Phosphorus Tests
Soil testing provides an indication of current soil levels, which allows agronomists and growers to determine the required rate of phosphorus to elicit a desired yield response under adequate growing conditions (McKenzie et al., 1995). As well, with multiple years of testing, soil testing indicates whether soil phosphorus is being depleted over time so that a grower can sustainably manage their soil resource (Grant and Flaten, 2019). Soil testing thus is a key part of sustainable phosphorus management.
Laboratories in Western Canada will use one of several soil phosphorus tests, with different tests being effective at different soil pH levels. As well, each method has a specific critical level, at which there is the greatest likelihood of a crop response, and below which, phosphorus is considered low.
Table 1 summarizes the pH levels and high and low values for the common soil phosphorus tests available in Western Canada. Tests using an anion exchange resin, such as the Plant Root Simulator (PRS) probes, can also be used to measure soil phosphorus available to plant roots (Ashworth and Mrazek, 1995; McKenzie et al.,1995; Qian et al., 1994; Karamanos, 2007). Soil test phosphorus concentration is usually given in parts per million (ppm), and can be converted to pounds acres (lb ac -1) in 15 centimetre (cm) depth soil increments by multiplying by 2. Another important conversion factor to note, especially when reviewing literature, is that fertilizer rates may be given as P or as P2O5. Typically, farmers are given fertilizer rates in lb P2O5 ac-1, whereas in research, rates are often given in kg P ha-1. To convert lb P ac-1 to lb P2O5 ac-1, multiply by 2.3. One final note on interpreting soil tests is that some soil tests will provide multiple available phosphorus tests. Look at the pH, or the buffer pH if provided, to determine the appropriate soil test to use.
Table 1. Soil pH and critical values for various soil phosphorus tests available in Western Canada (McKenzie et al. 1995)
Soil Test Method | Soil pH Range | Low Soil P | High Soil P |
ppm | |||
Olsen/Bicarb | ≥ 7.0 | < 10 | > 20 |
Bray 1 (weak) | ≤ 7.0 | < 15 | > 25 |
Bray 2 (strong) | ≤ 7.0 | < 27 | > 40 |
Kelowna/Modified Kelowna | Broad range | < 15 | > 30 |
C) Determining Fertilizer Phosphorus Rates
Every bushel produced requires a certain amount of phosphorus (Table 2). The full amount of phosphorus required per bushel of crop produced is known as the uptake rate. The amount of nutrients taken out of the field with harvested grain is known as the crop removal rate. Actual uptake is dependent on environmental conditions such as soil moisture and temperature, and also varies by specific crop variety (Johnston et al., 1999; Heard et al., 2006; Xie et al., 2017). Environmental conditions are highly variable and research into removal rates by variety is limited, thus, soil sampling at least every three years is recommended to ensure appropriate rates are being used to maintain or build soil phosphorus based on the desired outcome
Uptake and removal rates can be used to calculate phosphorus rates. Recommended application rates are often provided with soil tests to target specified yields but doing your own calculations of phosphorus balance is a good option to ensure you are targeting the desired outcome based on yield goals.
When using the long-term sustainability strategy with the aim of building soil phosphorus, it is difficult to determine an exact build rate, as the rate of build up will vary by growing conditions and with soil characteristics such as texture and pH (Grant et al., 2014; Mohr et al., 2016). A good strategy for determining a phosphorus build rate when soil levels are low would be to add an additional 20-30 lb P2O5 ac-1 above crop removal rates. Build rates must fit within the farm budget and equipment logistics. Continued soil testing is necessary to determine if rates are effectively building soil phosphorus.
D) Phosphorus Rate Response Research Results for Pulses
Pulse crops often do not respond strongly to fertilizer phosphorus, and instead scavenge native soil phosphorus as they can form AMF associations to acquire soil phosphorus, and are also able to release root exudates to acidify the root zone and solubilize phosphorus from calcium phosphate minerals. Although pulse crops do not always respond strongly to fertilizer phosphorus, fertilizer should be applied to prevent mining of soil phosphorus. Pulse crops will most likely respond to applied phosphorus in low phosphorus soils, in cold soils, in very wet or dry soil, or in rotation with non-AMF hosting crops such as canola (Schoenau presentation). Review of Western Canadian research specific to each pulse crop gives a good indication of what rates and conditions may produce a yield response.
Table 2. Pulse Crop Phosphorus Uptake & Removal Rates per Harvest Unit
Crop | Harvest Unit | Phosphorus Uptake (lb P2O5 per harvest unit) | Phosphorus Removal (lb P2O5 per harvest unit) | ||||
Low | High | Avg. | Low | High | Ave | ||
Peas | bushel | 0.76 | 0.92 | 0.84 | 0.62 | 0.76 | 0.69 |
Lentils | bushel | 0.73 | 0.90 | 0.82 | 0.60 | 0.66 | 0.63 |
Faba Beans | bushel | 0.70 | 0.85 | 0.78 | 0.67 | 0.78 | 0.73 |
Chickpeas | CWT | – | – | 0.83 | – | – | – |
Soybeans | bushel | 0.8 | 1.0 | 0.90 | 0.80 | 1.00 | 0.90 |
Dry Beans | CWT | – | – | 1.39 | – | – | 1.12 |
CWT = 100 pounds
Source: Adapted from Canadian Fertilizer Institute, 2001; Heard et al., 2006; and Government of Saskatchewan
Each pulse crop varies in their responsiveness to applied phosphorus. Faba beans may be highly responsive with some research reporting yields increasing at rates even above 80 lb P2O5 ac-1 (Henry et al., 1995; Holzapfel et al., 2017). Peas and lentils are considered intermediate in response with 15-30 lb P2O5 ac-1 most commonly eliciting a response when soils are low in phosphorus (less than 10 ppm Modified Kelowna phosphorus in the top 6 cm) (Henry et al., 1995, Karamanos et al, 2003, McKenzie et al., 2001, Knight et al 2012, Holzapfel et al., 2019). Soybeans, chickpeas, and dry beans respond variably to phosphorus application. Regardless of the responsiveness of the pulse crop to applied phosphorus, the amount used by the crop should eventually be replaced in the rotation to prevent soil depletion.
3) Right Timing
The highest demand for phosphorus in pulses is in the early season during the vegetative period and early reproductive phase, thus early season availability of phosphorus is essential to maximize crop yield potential. Saskatchewan research found that in peas and lentils, the highest uptake rate occurred at 28-49 days after emergence, which is around the time plants begin branching (Malhi et al., 2007). Due to the importance of early season phosphorus availability, application of some fertilizer close to the seed at the time of seeding is imperative. Newly developing plant root systems have limited surface area to access residual soil phosphorus. Without added phosphorus near the seed, juvenile roots may quickly deplete the phosphorus in their root zone and experience deficiency. Application of fertilizer phosphorus at seeding with early-sown pulse crops is especially important as cold soil temperature slows root growth and restricts the rate of phosphorus-releasing reactions and movement in soil, limiting plant root access to new soil phosphorus sources (Sheppard and Racz, 1984; Grant and Flaten, 2019). When seeding pulse crops first, application of phosphorus near the seed at seeding is an important practice to ensure early season availability.
Because pulse crops are effective scavengers of phosphorus, they will be able to utilize residual phosphorus applied in previous years. Another timing option is to apply excess phosphorus with the previous crop to cover removal rates for both crops, and to apply only the seed safe rate of starter phosphorus with the seed in the pulse year. This strategy would allow growers to achieve removal rates without compromising seed safety.
Applying phosphorus at the time of seeding is more effective than a foliar application, due to the importance of early season phosphorus for crop development. However, foliar phosphorus may be helpful as a top-up when a plant is exhibiting deficiency symptoms or is under stress with phosphorus limiting. Research in other regions found that foliar phosphorus did prove beneficial where soil levels were low or the crop was under stress (Benbella and Paulsen, 1998a; Benbella and Paulsen, 1998b; Girma et al., 2007; Mosali et al., 2006).
Research on low phosphorus Saskatchewan soils in peas compared 100% soil-applied, 100% foliar, and combinations of both (1:4 and 1:1 foliar to soil-applied phosphorus) at the 6-9 node stage. At a site that was highly phosphorus deficient, a 100% soil application and the combination treatments produced significantly higher yield than the 100% foliar treatment in one year. In the other site year, 100% seed-placed phosphorus was higher than all other treatments (Froese et al., 2018). This yield response is likely due to the importance of phosphorus availability early on in the season that is lacking when much or all is applied as a foliar application.
4) Right Placement
Due to the importance of early season phosphorus availability and its relatively low mobility in soil, banding is generally the best placement method for maximizing plant access and fertilizer use efficiency. Banding puts fertilizer phosphorus into the soil where moisture for dissolving fertilizer granules is higher in comparison to the soil surface, and where plant roots can access it early in the season (Grant et al., 2001). Because phosphorus moves so slowly in the soil, only a limited amount of broadcast phosphorus will likely make it to the rooting zone in the year of application. Incorporation of broadcast phosphorus through tillage will move the applied closer to the rooting zone, but will dry out soil.
Banding of phosphorus fertilizer increases fertilizer use efficiency, as higher broadcast rates are required to elicit the same yield response as a lower soil-placed rate (Grant and Flaten, 2019). In a Saskatchewan field study in the Brown soil zone, banding of 18 lb P2O5 ac-1 elicited a 30% yield increase for soybeans compared to phosphorus broadcast at up to 71 lb P2O5 ac-1 (Weiseth, 2015). As well, banding increases crop phosphorus uptake relative to weed uptake, whereas broadcast phosphorus provides nutrients non-selectively to both weeds and the crop (Blackshaw and Brandt, 2009; Blackshaw et al., 2004). Phosphorus use efficiency is also reduced with broadcasting because it can be lost through surface runoff, which presents a loss from the cropping system as well as a contaminant to watersheds (Wiens et al., 2019).
One appropriate use for broadcasting of phosphorus is to build up soil levels when using the long-term sustainability technique. Larger amounts of phosphorus can be applied by broadcasting than with seeding equipment, and as phosphorus is relatively immobile in soil, the nutrients will stay in the soil profile and build up soil levels. Incorporation is recommended to prevent losses from runoff (Grant and Flaten, 2019). Based on the above research, in most situations, phosphorus fertilizer should be banded rather than broadcast due to maximized plant uptake and use efficiency.
When banding phosphorus, depending on seeding equipment, a farmer may be able to apply fertilizer directly with the seed, in a sideband, or in a mid-row band. Fertilizer placed directly with the seed provides the seed with early season access to phosphorus, maximizing root growth and crop development. With banded fertilizer, the plant must rely on soil phosphorus for initial development until roots can reach the applied fertilizer. Banding may result in an early season deficiency, especially on low soil phosphorus and when the band is further from the seed (ex. mid-row band).
Top Dressing or Foliar Applications
A Saskatchewan study looking at foliar KH2PO4 applications compared to soil-applied phosphorus in peas as well as canola and wheat, found a better response to soil-applied than foliar because of improved early season phosphorus availability. Canola, having a larger leaf area and the most complete canopy closure at time of spraying, had greater uptake than wheat and peas, as it was able to capture more of the applied foliar phosphorus (Froese et al., 2018). In-crop topdressing of dry phosphorus fertilizer is not an effective placement method, as it has low mobility in the soil and will not move to the plant’s rooting zone in the year of application.
Seed Safety
Seed safety is an important component of fertilizer placement, as fertilizer can harm seed as a result of salt damage and ammonia toxicity (Grant and Flaten, 2019). Pulse crops can only tolerate a small amount of seed-placed phosphorus (Table 3).
Toxicity of seed-placed phosphorus to pulse crops is impacted by several factors related to seeding equipment, fertilizer source, and soil characteristics. The main variable in determining seedling phosphorus toxicity is the type of seeding equipment being used.
Seedling safety increases with narrower row spacing and wider openers, as the same amount of fertilizer is spread over a larger opener area, in more rows, in comparison to a seeding implement with wider row spacing and narrower openers. Seedbed utilization (SBU) is a calculation that indicates the area of seedbed over which the fertilizer will be spread.
Table 3. Safe Rates of Seed-Placed P2O5 for Pulse Crops
Crop | Saskatchewana (lb ac-1) | Manitobab (lb ac-1) |
---|---|---|
Faba Beans | 40 | 20 |
Dry Beans | 30 (pinto) | 10 |
Lentils | 20 | – |
Chickpeas | 20 | – |
Peas | 15 | 20 |
Soybeans | 20 (limited data) | 10 |
a Recommendations from Saskatchewan Ministry of Agriculture, based on 1″ openers with 9″ row spacing (10-15% SBU) and good-to-excellent moisture conditions, using MAP
b Recommendations from Manitoba Agriculture based on disc or knife openers with 1” spread and 6-7” row spacing (15% SBU)
Fertilizer source also has an impact on seed safety. DAP (18-46-0) has reduced seed safety in comparison to MAP (11-52-0), as DAP produces higher ammonia toxicity. TSP (0-45-0) has a lower impact on pulse seeds due to the absence of nitrogen and a lower salt index, but is not as widely available (McKenzie et al., 2001). Seed safety guidelines were developed using MAP fertilizer, thus agronomists and growers should take into consideration the phosphorus source being used when determining the amount to put with the seed. Polymer- coated phosphorus products may also improve seed safety by slowing the dissolution of the fertilizer.
Soil factors impacting phosphorus toxicity include soil moisture, texture, organic matter content, and pH. Seedling damage from phosphorus fertilizer will be worse on dry, coarse textured soils at a higher pH. In moist soils, fertilizer concentration in the soil solution is lower than in dry soils. Coarse textured and low organic matter soils have a lower CEC and thus fewer exchange sites to adsorb ammonium, allowing more ammonium in the soil solution to convert to ammonia and damage seed (Karamanos et al., 2003). Similarly, higher pH shifts equilibrium towards ammonia rather than ammonium increasing ammonia toxicity (Kaiser and Rubin, 2013; Rehm and Lamb, 2009). Several factors that vary by farm and soil type impact the safety of seed-placed fertilizer. These factors must be considered to determine whether the government seed safety guidelines are too high or low for the specific crop to be grown.
Seed safe rates of phosphorus are well below crop removal rates for most pulse crops. In order to apply removal rates, phosphorus beyond seed safe levels must be applied in the sideband or mid-row, broadcast and incorporated, banded ahead of time, or applied with the previous crops.
Summary
- Right Source—in a form that suits the use; for immediate uptake by the crop phosphorus needs to be in a readily
available form; for soil building or longer term sustainability the source of phosphorus is less critical - Right Rate— the rate that will supply enough phosphorus (along with soil phosphorus) for the crop to reach target yields; removal rates are good rates for sustainability over the long term; soil testing for monitoring soil levels over time is important
- Right Timing— Phosphorus needs to be available for uptake throughout the season but highest requirements are during rapid vegetative growth and reproductive phases.
Applications early in the season (seeding) target availability during growth - Right Placement—Phosphorus does not move in the soil and needs to be accessible to the roots. Banding into the soil is the best option and can include banding prior to seeding, placing with the seed, sidebanding, and/or mid-row banding
Phosphorus is an essential nutrient for pulse crop production, as it is a component of many plant molecules necessary for growth and development, as well as for nitrogen fixation. 4R stewardship of phosphorus in pulse crops involves using the right source, at the right rate, applied at the right time, in the right place. Understanding the 4Rs for phosphorus, taking into consideration its high reactivity and low mobility in the soil, and the importance of early season phosphorus nutrition for plants, is essential to maximize pulse crop production.
The right source of phosphorus for pulse crops is the source that will be available to meet plant demands with the least amount of environmental impact. The most readily available form of phosphorus is commercial fertilizer, with dry monoammonium phosphate (MAP) and liquid ammonium polyphosphate (APP) being the most common sources available in Western Canada. Using by-product forms of phosphorus, such as manure and struvite, is an effective means to recycle phosphorus. Fertilizer additives and coatings, and microbial products have sometimes shown inconsistent results in improving phosphorus uptake and yield in pulses. The right rate of phosphorus for pulse crops is crop and field specific and depends on consideration of target yields and soil availability. Pulse crops show variable responses to phosphorus fertilization in the year of application, with the greatest likelihood of response on soils that test low in supplies of plant available phosphorus.
To maintain or build soil phosphorus levels, removal rates should be considered over time. The greatest demand for phosphorus is in the early season, thus the right timing for pulse crops, especially when soil phosphorus is low, is at seeding.
Alternatively, higher rates can be applied in the year prior to a pulse, as pulse crops effectively scavenge residual soil phosphorus. The right placement for phosphorus is in-soil, considering maximum safe rates with the seed, and proximity from the seed-row. Soil application of phosphorus maximizes plant uptake and nutrient use efficiency in comparison to broadcasting. Seeding equipment, fertilizer source, and soil conditions impact seed safety and are important factors to consider when seed placing phosphorus. As well, seed safe rates are often lower than crop removal rates, thus more phosphorus should be applied through another placement method to maintain or build soil levels. 4R stewardship of phosphorus in pulse crops benefits both the environment, and farm bottom line, as the most agronomically beneficial practices of 4R phosphorus management are also the most environmentally sustainable.
References
- Ackerman, J. N., F. Zvomuya, N. Cicek, and D. Flaten. 2013. Evaluation of manure derived struvite as a phosphorus source for canola. Can. J. Plant Sci. 93:419-424.
- Ashworth, J. and K. Mrazek. 1995. “Modified Kelowna” test for available phosphorus and potassium in soil. Commun. Soil Sci. Plan. 26(5-6):731-739.
- Bekele, T., B. Cino, P. Ehlert, A. Van der Maas, and A. Van Diest. 1983. An evaluation of plant-borne factors promoting the solubilization of alkaline rock phosphates. Plant Soil 75(3):361-378.
- Benbella, M., and G.M. Paulsen. 1998a. Efficacy of treatments for delaying senescence of wheat leaves: I. Senescence under controlled conditions. J. Agron. 90(3):329- 332.
- Benbella, M. and G.M. Paulsen. 1998b. Efficacy of treatments for delaying senescence of wheat leaves: II. Senescence and grain yield under field conditions. J. Agron. 90(3):332-338.
- Blackshaw, R. E., and R.N. Brandt. 2009. Phosphorus fertilizer effects on the competition between wheat and several weed species. Weed Biol Manag. 9(1):46-53.
- Blackshaw, R.E., L.J. Molnar, and H.H. Janzen. 2004. Nitrogen fertilizer timing and application method affect weed growth and competition with spring wheat. Weed Sci. 52(4):614-622
- Bouldin, D.R., and E.C. Sample. 1959. Laboratory and greenhouse studies with monocalcium, monoammonium, and diammonium phosphates. Soil Sci. Soc. Am. J. 23(5):338-342.
- Close, D.C., and C.L. Beadle. 2003. The ecophysiology of foliar anthocyanin. Bot. Rev. 69(2):149-161.
- Du, C., Z. Jian-ming, and A. Shaviv. 2006. Release characteristics of nutrients from polymer-coated compound controlled release fertilizers. J Polym Environ. 14:223-230.
- Froese, S.R.E. 2018. Response of canola, wheat and pea to foliar phosphorus fertilization in three Saskatchewan soil zones. M. Sc. Thesis, University of Saskatchewan, Saskatoon, SK.
- Gan, Y., F. Selles, K.G. Hanson, R.P. Zentner, B.G. McConkey, S.V. Angadi, and C.L. McDonald. 2003. Rhizobium Inoculant Formulation and Placement in Lentil and Chickpea in the Semiarid Canadian Prairies. In Proc. (CD-ROM) Soils and Crops Workshop 2003, Saskatoon, SK. Feb 17-18, 2003.
- Girma, K., K.L. Martin, K.W. Freeman, J. Mosali, R.K. Teal, W.R. Raun, S.M. Moges, and D.B. Arnall. 2007. Determination of optimum rate and growth stage for foliarapplied phosphorus in corn. Commun. Soil Sci. Plan. 38(9- 10):1137-1154
- Gonzalez Ponce, R., and M.E. Garcialopez. 2007. Evaluation of struvite as a fertilizer: A comparison with traditional P sources. Agrochimica-Pisa. 51(6):301-308.
- Government of Saskatchewan. Phosphorus fertilization in crop production. https://www.saskatchewan.ca/business/agriculture-natural-resources-and-industry/agribusiness-farmers-and-ranchers/crops-and-irrigation/soils-fertility-and-nutrients/phosphorus-fertilization-in-crop-production. Available online (accessed April 13, 2020)
- Government of Saskatchewan. Guidelines for safe rates of fertilizer applied with the seed. https://www.saskatchewan.ca/business/agriculture-natural-resources-and-industry/agribusiness-farmers-and-ranchers/crops-and-irrigation/soils-fertility-and-nutrients/guidelines-for-safe-rates-of-fertilizer-applied-with-the-seed. Available online (accessed April 13, 2020)
- Grant, C. A. 2002. Effect of coated and non-coated monoammonium phosphate fertilizer on phosphorus uptake, crop yield and nutrient profile of wheat and canola. Pages 13. Agriculture and Agri-Food Canada, Brandon, MB.
- Grant, C.A., L.D. Bailey, J.T. Harapiak, and N.A. Flore. 2002. Effect of phosphate source, rate and cadmium content and use of Penicillium bilaii on phosphorus, zinc, and cadmium concentration in durum wheat grain. J. Sci. Food Agric. 82:301–308. doi:10.1002/jsfa.1034
- Grant, C., S. Bittman, M. Montreal, C. Plenchette, and C. Morel. 2005. Soil and fertilizer phosphorus: Effects on plant P supply and mycorrhizal development. Can. J. Plant Sci. 85:3–14.
- Grant, C.A., and D. Flaten. 2019. 4R Management of Phosphorus Fertilizer in the Northern Great Plains: A Review of the Scientific Literature. Available online: https://fertilizercanada.ca/wp-content/uploads/2019/07/4R-P-fertilizer-mgmt-for-NGP-Detailed-version-July-3-2019_VF.pdf
- Grant, C. A., D.N. Flaten, D.J. Tomasiewicz, and S.C. Sheppard. 2001. The importance of early season phosphorus nutrition. Can. J. Plant Sci. 81:211–224.
- Grant, C.A., A.R.S. Hosseini, D. Flaten, O. Akinremi, O. Obikoya, and S. Malhi. 2014. Change in availability of phosphorus, cadmium and zinc applied in monoammonium phosphate after termination of fertilizer application. In: 20th World Congress of Soil Science, Jeju, Korea. 8–14 June. IUSS, Vienna.
- Grenkow, L.A. 2013. Effect of seed-placed phosphorus and sulphur fertilizers on canola plant stand, early season biomass and seed yield. M. Sc. Thesis, University of Manitoba, Winnipeg, MB.
- Heard, J, B. Brolley, and R. Park. 2006. Nutrient Uptake and Removal Values for Dry Beans, Soybeans and Sunflowers in Manitoba. Unpublished Report to Manitoba Soil Fertility Advisory Committee, March 17, 2006.
- Henry, J.L., A.E. Slinkard, and T.J. Hogg. 1995. The effect of phosphorus fertilizer on establishment, yield and quality of pea, lentil and faba bean. Can. J. Plant Sci. 75:395-398.
- Holzapfel, C. 2015. Phosphorus fertilization and fungicide effects on faba bean establishment and yield. Annual Report for the Agricultural Demonstration of Practices and Technologies (ADOPT) Program.
- Holzapfel, C. 2017a. Developing phosphorus management recommendations for soybeans in Saskatchewan. Project Report for the Saskatchewan Pulse Crop Development Board.
- Holzapfel, C., G. Hnatowich, M. Hall, J. Pratchler, J. Weber, and B. Nybo. 2019. Enhanced fertilizer management for optimizing yield and protein in field pea. Annual Report to Saskatchewan Pulse Growers.
- Hopkins, B. G. 2015. Phosphorus. Pages 65 -126 in A. V. Barker, D. J. Pilbeam, eds. Handbook of plant nutrition. CRC press, Boca Ratan, FL.
- Hopkins, B. G., K.J. Fernelius, N.C. Hansen, and D.L. Eggett. 2018. AVAIL phosphorus fertilizer enhancer: Meta-Analysis of 503 field evaluations. J. Agron. 110(1):389-398.
- Hoppo, S.D., D.E. Elliott, and D.J. Reuter. 1999. Plant tests for diagnosing phosphorus deficiency in barley (Hordeum vulgare L.). Aust. J. Exp. Agric. 39(7):857-872.
- Johnston, A.M., S.S. Malhi, J. Schoenau, and S. Exner. 1999. Biomass accumulation and nutrient uptake by annual crops. Research project report to the Canadian Fertilizer Institute, Ottawa, ON.
- Johnston, A.E., P.R. Poulton, P.E. Fixen, and D. Curtin. 2014. Phosphorus: its efficient use in agriculture. Pages 177-228 Adv. Agron. Elsevier.
- Johnston, A.E., and I.R. Richards. 2003. Effectiveness of different precipitated phosphates as phosphorus sources for plants. Soil Use Manage. 19(1):45-49.
- Kaiser, D.E. and J.C. Rubin. 2013. Maximum rates of seed placed fertilizer for corn for three soils. J. Agron. 105(4):1211-1221
- Karamanos, R. 2007. Agroeconomics of phosphate fertilizer in Manitoba. In: Proceedings of the 8th Annual Manitoba Agronomists Conference. 11–12 Dec. 2007. University of Manitoba, Winnipeg, MB, Canada.
- Karamanos, R.E., N.A. Flore, and J.T. Harapiak. 2003. Response of field peas to phosphate fertilization. Can. J. Plant Sci. 83:283–289.
- Karamanos, R.E., N.A. Flore, and J.T. Harapiak. 2010. Re-visiting use of Penicillium bilaii with phosphorus fertilization of hard red spring wheat. Can. J. Plant Sci. 90(3):265-277.
- Kastens, T., K. Dhuyvetter, J. Schmidt, and W. Stewart. 2000. Wheat yield modeling: How important is soil test phosphorus? Better Crops 84(2):8-10.
- Knight, J. D. 2011. Soil activity of P solubilizing microorganisms. Report to Saskatchewan Agricultural Development Fund. Pages 38 pp. University of Saskatchewan, Saskatoon, SK
- Knight, J.D. 2012. Importance of P nutrition on N2 fixation, nutrient uptake and productivity of pea. Report to Saskatchewan Agricultural Development Fund. Pages 58 pp. University of Saskatchewan, Saskatoon, SK
- Liu, J., Y. Hu, J. Yang, D. Abdi, and B.J. Cade-Menun. 2015. Investigation of soil legacy phosphorus transformation in long-term agricultural fields using sequential fractionation, P K-edge XANES and solution P NMR spectroscopy. Environ. Sci. Technol. 49(1):168-176.
- Liu, J., Y. Hu, J. Yang, D. Abdi, and B.J. Cade-Menun. 2015. Investigation of soil legacy phosphorus transformation in long-term agricultural fields using sequential fractionation, P K-edge XANES and solution P NMR spectroscopy. Environ. Sci. Technol. 49(1):168-176.
- Massey, M.S., J.G. Davis, R.E. Sheffield, J.A. Ippolito. 2007. Struvite production from dairy wastewater and its potential as a fertilizer for organic production in calcareous soils. In: International Symposium on Air Quality and Waste Management for Agriculture. CD-Rom Proceedings of the 16–19 September 2007,Conference (Broomfield, Colorado), USA. ASABE Publication Number 701P0907cd
- Malhi, S.S., A.M. Johnston, J.J. Schoenau, Z.H. Wang, and C.L. Vera. 2007. Seasonal Biomass Accumulation and Nutrient Uptake of Pea and Lentil on a Black Chernozem Soil in Saskatchewan. J. Plant Nutr. 30:721-737
- Malhi, S., M. Nyborg, D. Penney, L. Kryzanowski, J. Robertson, and D. Walker. 1993. Yield response of barley and rapeseed to P fertilizer: Influence of soil test P level and method of placement. Commun. Soil Sci. Plant Anal. 24:1–10. doi:10.1080/00103629309368777
- McKenzie, R.H., E. Bremer, L. Kryzanowski, A.B. Middleton, E.D. Solberg, D. Heaney, G. Coy, and J. Harapiak. 2003. Yield benefit of phosphorus fertilizer for wheat, barley, and canola in Alberta. Can. J. Soil Sci. 83:431–441. doi:10.4141/S02-078
- McKenzie, R.H., L. Kryzanowski, K. Cannon, E. Solberg, D. Penney, G. Coy, D. Heaney, J. Harapiak, and N. Flore. 1995. Field evaluation of laboratory tests for soil phosphorus. Alberta Agricultural Research Institute, Edmonton, AB, Canada
- McKenzie, R.H., A.B. Middleton, E.D. Solberg, J. DeMulder, N. Flore, G.W. Clayton, and E. Bremer. 2001. Response of pea to rate and placement of triple superphosphate fertilizer in Alberta. Can. J. Plant Sci. 81:645–649. doi:10.4141/P01-007
- McKenzie, R., J. Stewart, J. Dormaar, and G. Schaalje. 1992a. Long-term crop rotation and fertilizer effects on phosphorus transformations: I. In a Chernozemic soil. Can. J. Soil Sci. 72:569–579. doi:10.4141/cjss92-047
- McKenzie, R., J. Stewart, J. Dormaar, and G. Schaalje. 1992b. Long-term crop rotation and fertilizer effects on phosphorus transformations: II. In a Luvisolic soil. Can. J. Soil Sci. 72:581–589. doi:10.4141/cjss92-048
- Miller, M., C. Mamaril, and G. Blair. 1970. Ammonium effects on phosphorus absorption through pH changes and phosphorus precipitation at the soil-root interface. J. Agron. 62(4):524-527.
- Mohr, R.M., C.A. Grant, G.R. Bardella, and D.N. Flaten. 2016. Final report: Long-term management of phosphorus fertilizer to maximize yield and minimize cadmium in soybeans under Manitoba conditions (Experiment B of the project ‘Phosphorus P Fertilizer Rates page 48 Fertilization Beneficial Management Practices for Soybeans in Manitoba’). Pages 14. Agricuture and Agri-Food Canada, Brandon, MB.
- Mohr, R., B. Irvine, C, Grant, C. Holzapfel, T. Hogg, S. Malhi, and A. Kirk. 2013. Response of canola to the application of phosphorus fertilizer and Penicillium bilaii (JumpStart®). Page 24. Saskatchewan Canola Development Commission, Saskatoon, SK
- Monreal, M.A., C.A. Grant, R.B. Irvine, R.M. Mohr, D.L. McLaren, and M. Khakbazan. 2011. Crop management effect on arbuscular mycorrhizae and root growth of flax. Can. J. Plant Sci. 91(2):315-324.
- Mosali, J., K. Desta, R.K. Teal, K.W. Freeman, K.L. Martin, J.W. Lawles, and W.R. Raun. 2006. Effect of foliar application of phosphorus on winter wheat grain yield, phosphorus uptake, and use efficiency. J. Plant Nutr. 29(12):2147-2163.
- Nielsen K.L., A. Eshel, and J.P. Lynch. 2001. The effect of phosphorus availability on the carbon economy of contrasting common bean (Phaseolus vulgaris L.) genotypes. J. Exp. Bot. 52:329-339.
- Niu Y.F., R.S. Chai, G.L. Jin, H. Wang, C.X. Tang, and Y.S. Zhang YS. 2012. Responses of root architecture development to low phosphorus availability: a review. Ann. Bot. 112:391–408.
- Ozanne, P. G. 1980. Phosphate nutrition of plants – A general treatise. pp. 559-589. In: Khasawnet, F.E., E.C. Sample, and E.J. Kamprath. The Role of Phosphorus in Agriculture. American Society of Agronomy.
- Qian, P., J.J. Schoeau, and R.E. Karamanos. 1994. Simultaneous extraction of available phosphorus and potassium with a new soil test: A modification of Kelowna extraction. Comm. Soil Plant. Anal. 25(5-6):627-635.
- Rahman, M.M., M.A.M. Salleh, U. Rashid, A. Ahsan, M.M. Hossain, and C.S. Ra. 2014. Production of slow release crystal fertilizer from wastewaters through struvite crystallization – A review. Arab. J. Chem. 7:139-155.
- Rehm, G.W., and J.A. Lamb. 2009. Corn response to fluid fertilizers placed near the seed at planting. Soil Sci. Soc. Am. J. 73(4):1427-1434.
- Rotaru, V., and T.R. Sinclair. 2009. Interactive influence of phosphorus and iron on nitrogen fixation by soybean. Environ. Exp. Bot. 66(1):94-99.
- Sandana, P., and D. Pinochet. 2014. Grain yield and phosphorus use efficiency of wheat and pea in a high yielding environment. J. Soil Sci. Plant Nutr. 14(4):973-986.
- Sarker B.C., P. Rashid, and J.L. Karmoker. 2015. Anatomical changes of lentil (lens culinaris medik.) under phosphorus deficiency stress. Bangladesh J. Bot. 44(1):73-78.
- Schoenau, J. J., P. Qian, and T. King. 2007. Strategies for improving the efficiency and crop safety of starter fertilizer phosphorus and potassium. Pages 23. University of Saskatchewan, Saskatoon.
- Sheppard, S. C., and G.J. Racz. 1984a. Effects of soil temperature on phosphorus extractability I. Extractions and plant uptake of soil and fertilizer phosphorus. Can. J. Soil Sci. 64:241–254.
- Syers, J., A. Johnston, and D. Curtin. 2008. Efficiency of soil and fertilizer phosphorus use. FAO Fertilizer and Plant Nutrition Bulletin No. 18.(FAO: Rome).
- Vessey, J. K. and K.G. Heisinger. 2001. Effect of Penicillium bilaii inoculation and phosphorus fertilisation on root and shoot parameters of field-grown pea. Can J. Plant Sci. 81(3):361-366
- Weiseth, B. 2015. Impact of fertilizer placement on phosphorus in crop, soil, and run-off water in a Brown Chernozem in South-Central Saskatchewan. M. Sc. Thesis, University of Saskatchewan, Saskatoon, SK.
- J. Wiens, B. Cade-Menun, B. Weiseth and J.J. Schoenau, 2019. Potential Phosphorus Export in Snowmelt as Influenced by Fertilizer Placement Method in the Canadian Prairies. Journal of Environmental Quality 48:586-593.
- Xavier, L.J.C., and J.J. Germida. 1997. Growth response of lentil and wheat to Glomus clarum NT4 over a range of P levels in a Saskatchewan soil containing indigenous AM fungi. Mycorrhiza 7(1):3-8
- Xie, J., J. Schoenau, and T.D. Warkentin. 2017. Yield and uptake of nitrogen and phosphorus in soybean, pea, and lentil and effects on soil nutrient supply and crop yield in the succeeding year in Saskatchewan, Canada. Can. J. Plant Sci. 98(1):5-16
- Zhang, Z., H. Liao, and W.J. Lucas. 2014. Molecular mechanisms underlying phosphate sensing, signaling, and adaptation in plants. J. Intr. Plant Biol. 56(3);192–220